The New Cancer Models, Part 2: A Fly of One's Own
By Aaron Krol
We know that cancer is a personal disease, with each case displaying a unique genetic makeup that demands uniquely targeted therapy. Yet even as private companies like Foundation Medicine rush to capitalize on personalized cancer treatment, the basic research that supports these efforts still relies on flawed models: animal cancers, or human cancer cell lines, which may not map appropriately to live human tumors at all, let alone to a specific patient’s disease. This week, Bio-IT World is taking a look at three groups who are trying to update cancer models for modern drug discovery with a personal touch.
December 5, 2013 | If you visit the laboratory of Dr. Ross Cagan, on the 25th floor of the Annenberg Building at the Mount Sinai School of Medicine, and you ask very nicely, you just might get a chance to meet a Mark Beeninga fly. Mark was the first patient enrolled at the Mount Sinai Center for Personalized Cancer Therapeutics (CPCT), and like all humans, he shares about 60% of his DNA with Drosophila melanogaster, the common fruit fly.
Unless you’re looking at Mark’s tumor, and at this particular tribe of fruit flies. They share just a tiny bit more.
Specifically, they share the particular set of genes that is making them both sick. When Mark arrived at Mount Sinai, he had a biopsy taken of his tumor, and that tissue sample was sent to the Icahn Institute for Genomics and Multiscale Biology. There, renowned geneticist Dr. Eric Schadt and his team sequenced the tumor’s DNA and came up with an exhaustive list of the 100-200 unique mutations that distinguish this population of cancer cells from Mark’s healthy tissue. From this detailed cancer profile, a personally tailored fruit fly could be made with the same disease Mark has.
CPCT is sort of an advanced program for particularly gifted cancers. Patients with tumors that aren’t responding to traditional treatments go there in the hopes of finding a custom-made cure. Currently CPCT is accepting patients with medullary thyroid cancer, although early enrollees have also had colon cancer or triple-negative breast cancer, and the program will shortly be expanding to generally admit these cases as well. Medullary thyroid cancer is not exceptionally lethal, by cancer standards. The five-year survival rate is upwards of 80%, partly thanks to the effectiveness of simple surgical interventions, which in the best cases can cure the patient outright.
In the worst cases, however – like Mark’s – the cancer has already spread to the lymph nodes by the time a diagnosis is made. Now the prognosis is poorer and the interventions more difficult. Radiation therapy is almost always recommended, but ideally a well-targeted drug cocktail will address the specific mutations that have caused the patient’s thyroid cells to grow out of control. In most institutions, it would be considered state-of-the-art at this point to sequence a tumor’s suite of unique genes, and start searching the literature for drug associations that have been discovered through trials on cancer cell lines or animal models. Most institutions don’t have the equipment to do that much.
At CPCT, that’s just the starting point.
The Empirical Approach
A good cancer model is hard to come by. For some types of cancer, there are dozens or even hundreds of cancer cell lines used around the world to test drug responses. A few of these will contain reasonably close matches with certain patients’ specific cancer genes, but the chances of an exact match are astronomically improbable, while the chances of wild divergence are quite high. Some cell lines have picked up strange and novel mutations in their years of independent existence, and no longer closely resemble any human cancer. Cancer cell lines aren’t used for testing a specific patient’s ideal therapy, but if you’re lucky they may have been used to produce some solid research indicating that a tumor with a given mutation is likely to respond to a given drug.
A perfect cancer model is pretty easy to imagine. It would be the tumor itself, somehow divorced from the patient’s body and transplanted to the lab. Some researchers are working on making this a reality – see the previous entry in this series – but for now this technology lies well in the future.
But a great cancer model, something that a well-equipped laboratory could make right now to bring a strong analog to a specific patient’s cancer onto the workbench – that’s up for a certain amount of debate. No one has the capability to reliably transplant hundreds of genes into a model organism, so you have to ask how many are really important. Certainly it’s more than just one or two core driver mutations: if knowing whether a medullary thyroid cancer is a RET- or a RAS-mutant were enough to choose an effective drug course, silver bullet therapies would already be a reality. But which cancer genes you can safely dispose of, and whether they’ll be enough to make a tailored animal model practical, is anyone’s guess.
Dr. Cagan hates guessing. A typical lab with access to a tumor’s genetic code, he told Bio-IT World in an interview at his Mount Sinai office overlooking uptown Manhattan, will “look for commonalities between patients, and try to decide from that what’s a driver [gene] and what’s a passenger… What’s different about our approach is that we don’t make any assumptions.”
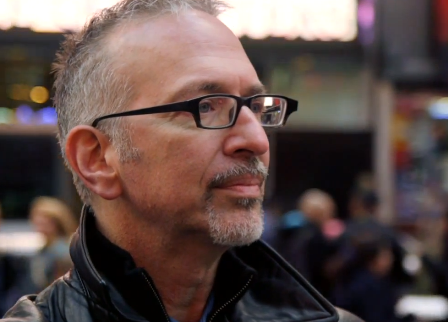
Ross Cagan is one of CPCT’s three directors, along with Dr. Schadt and Dr. Marshall Posner. His goal is to make a fruit fly with all of a patient’s most crucial mutations intact, and like a true Drosophila enthusiast, he figures if he’s breeding one line of fruit flies, he might as well breed dozens. Having identified a tumor’s base mutation, his lab takes a pre-existing fruit fly with that variant, and adds every single other variant Eric Schadt’s team detected in the cancer to make a massive matrix of two-hit flies. “One by one by one, we just test them functionally in the base fly,” he says. Most of the two-hit flies will show statistically identical symptoms to the simple base fly, and their mutations are discarded. But a few will have measurably more or less tumor growth. Their mutations – no matter if there’s no independent evidence to link those variants to cancer – go into the final stew. “Even if this particular gene is mutated only in this patient, and no one else in the world,” says Dr. Cagan, “we capture it.”
Cagan and his colleagues call this the empirical approach. The layperson might know it better as the shotgun method. But flies are cheap, and they grow fast, and the upshot of this process is a comprehensive list of the mutations in a patient’s cancer cells that affect tumor growth in flies. The Cagan lab has generally found somewhere between eight and twelve genes to be significant in a given case, but if it were twenty-five, they would know that too.
From here Cagan turns things over to Peter Smibert, a senior scientist in the lab, whose specialty is gene transfer. Eight to twelve genes may not sound like a lot, but it’s a rare feat to insert so many novel mutations into an organism’s genome in such a way that you can track their location in the genome and their level of expression in individual cells. For this, Smibert builds a single plasmid with RNA interference triggers for every gene on the list, suppressing these genes’ expression. This whole complex of suppressors can then be inserted at a specific site on a specific fly chromosome. “This vastly simplifies the genetics,” says Smibert, “so that we can do downstream tests with this. By just having one single transgene in a predictable spot in the genome, we can combine it with other transgenes we need to express it, to mark the cells in which it’s expressed, and follow what’s going on.”
In this way, the lab creates a population of cancerous flies custom-made for each patient in the CPCT program. The whole process takes about one to two weeks, and it will only get easier as time goes on. “The plan is to have a bunch of off-the-shelf models,” says Smibert, which have complexes of the known drivers built in already. That way, only the most unique active mutations have to be added to the mix. “So the hope is there’s no delay. As soon as we know the sequence of the patient, we can say, okay, they fit into this base model.”
Why Fly?
Flies aren’t perfect models for human cancer. When it comes to treating a single patient, a personalized fly is much better than a generic cell line, but the lineages that gave rise to fruit flies and human beings diverged over half a billion years ago, and their genomes have not kept in touch. “There are a few well-known cancer driver genes that don’t appear to exist in flies,” admits Smibert. Occasionally he will have to substitute another cancer gene in a relevant pathway when no true ortholog exists, although he tries very hard to avoid this. Some types of cancer – say, prostate cancer, or estrogen-dependent breast cancer – will probably never be amenable to fly models, because the relevant pathways just aren’t present in the insect anatomy.
“Mainly I would say the details are going to be different, and details can matter,” says Dr. Cagan. “But on the flip side, they have most of the major organs we have, so the whole-body experience is there… They have basically all the major signaling pathways that we have – the majority were actually named in flies – and so when you want to build solid tumors, and you want to make complex models, flies are basically your only choice. I can’t imagine another model where you can get a good, solid tumor, and make an eight-hit model out of it, and then screen it.”
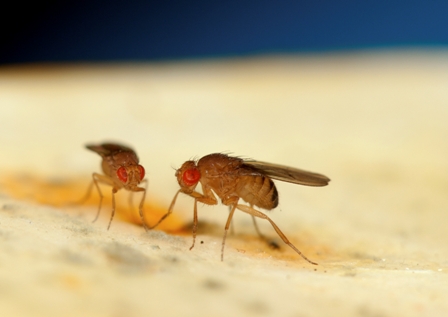
The screening step is key. Mount Sinai is perfectly capable of breeding PDX (patient-derived xenograft) mice with live human tumors implanted, and in fact CPCT has plans to incorporate PDX mice at other stages of the exploratory process. But they can’t make hundreds of these, and they can’t make them quickly. PDX mice would be appropriate if you had a good guess as to what drug therapy might work for a patient and wanted to do a practice run – but again, Dr. Cagan is not a guesser.
With hundreds of personalized flies, breeding naturally and at virtually no cost in the lab, he can bring his empirical approach to choosing the right drug combination for a patient’s individual cancer. This time, the shotgun in the shotgun method is a robotic system with 96 workcells, in which are placed both flies and compounds randomly selected from the full list of FDA-approved pharmaceuticals – in the neighborhood of a thousand drugs altogether.
Cagan isn’t looking for a silver bullet. What he wants is any miscellaneous cocktail that will reduce the tumors in his flies. He’s a strong proponent of polypharmocology, the notion that how drugs behave in tandem isn’t reliably predicted by how they behave alone. “If a single drug could work,” he says, “we wouldn’t be having this conversation… We actually assume it’s going to take at least two drugs, and in fact we’re talking about one patient now who’s probably going to go to three drugs.”
Personalized flies make this speculative program much more sensitive than other drug screens on cancer models. Plenty of compounds have made it to patients after curing cancer in mice with single driver mutations, only to fizzle in clinical trials. “As the genetic complexity of our tumor models goes up, the resistance to drugs goes up,” says Cagan. “So we’re seeing why these simple one- and two-hit mice have not been very predictive, because as soon as we step up into three and four, drugs that failed in the marketplace fail in those flies, even though they work beautifully in the one-hit flies.”
The CMCT process for drug screening is similar to the creation process for the flies themselves. At first, Cagan wanted to try every possible two-drug combo, but time limitations prohibit testing each of the hundreds of thousands of variables involved. So instead his lab screens each drug individually, keeps any compound that shows an effect alone, and then tries the matrix of each of those drugs in combination.
They don’t prefer cancer drugs in particular. “If you look at people who have done empirical screens,” says Cagan, “they typically come up with antipsychotics and things like that for cancer. All we know with these drugs is they’re biologically active.” For that matter, the only thing special about the FDA-approved list is that Mount Sinai is allowed to administer those drugs to patients if Cagan comes up with a hit. Before the CMCT program was a reality, the lab used much the same process for novel drug discovery.
“At this point we’re really standing on the shoulders of work that’s been done in the lab for the last four or five years,” says Smibert. “Especially by a couple of the postdocs, Tirtha [Das] and Erdem [Bangi].” This team’s work, on fruit flies with multiple mutations but no direct patient counterparts, has led to publications in Nature and Cell demonstrating novel compounds’ efficacy in treating cancer in mice.
The same group has also run earlier polypharmocological screens on multiple-mutant flies, yielding valuable insights for CPCT’s work with real patients. For one thing, the lab has become less focused on “clean” compounds that shut down gene expression altogether. “When we throw the libraries at these flies, and just see what works, we basically never get clean drugs,” says Cagan. “We always get these low-specificity drugs that hit a bunch of stuff, and then we need to go figure out what in the world they’re hitting… So that was really a lesson, that maybe we shouldn’t obsess over targets. Maybe we should obsess over drugs.”
In one case, the lab’s prior work provided a more direct lesson. One of CPCT’s first patients – before the program was officially launched – was Stephanie Lee, who was suffering from a difficult-to-treat case of colon cancer. Her tumor happened, just by coincidence, to be a close match to a fly line the lab had created on its own before personalized flies were even imagined. This helped them quickly come up with a drug cocktail they could recommend in Stephanie’s case.
And the CPCT fly program could easily cycle right back to the drug discovery it began with. At this point, Cagan’s team has about twenty lines of patient-derived flies, which, with their minimal upkeep costs, will still be bred long after the patients have left. These tailor-made insects can then be put in rotation in any lab looking for a good, multiple-gene cancer model based on real human cases.
Not Just Personalized. Personal
Few of the leading members of the CPCT are accustomed to working with patients. Dr. Schadt, for instance, came to Mount Sinai in 2011 from the pharmaceutical industry. Dr. Cagan is a fly guy, who is also working with Drosophila diabetes models. But they’ve had a lot of help adjusting to their new roles attached to the clinic – not just from Mount Sinai, but also from the patients themselves.
CPCT takes a community-minded approach to cancer care, and wants to provide a dedicated space for patient feedback. It’s an opportunity Mark Beeninga has been taking advantage of, blogging on the skeletal CPCT website, mountsinaicpct.org, about his disease and treatment. “Talking to Mark has actually been very enlightening, and caused us to focus more on things like toxicity, which frankly I hadn’t really concerned myself with,” says Cagan. Now his lab takes note of which compounds cause more toxic responses, and takes that into account when choosing between different cocktails that have shown effectiveness in curing their flies. There are plans in the works to add PDX mice to the mix for a final round of screening that will provide better information on toxicology.
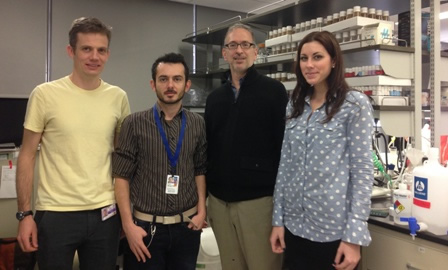
“So another aspect of the center is really trying to connect to the patients,” continues Cagan, “and getting them to tell us what they need.” For their part, the patients sometimes see their enrollment in the Center as an opportunity to help patients like themselves. CPCT respects patients’ wishes concerning their personal data, but to some, like Mark, data isn’t personal at all: it’s a resource to be widely shared with anyone who could benefit.
“[Mark] wants everything to be released,” says Cagan, “and in fact I have been already discussing it with clinicians as per his request. We actually hope to publish his data and put it out there. In fact, that was almost his criteria for coming into the Center.” That information will go toward new research exploring exactly why the cocktails Cagan’s lab comes up with for Mark are able to target his cancer. Hopefully, future patients with similar mutations will have a head start in finding a suitable therapy, and new leads will be generated for drug discovery.
For now, CPCT is taking it one patient at a time. Their methods may seem chaotic, but after growing dozens of fly lines and testing hundreds of drug combinations, the lab can bring forward empirically substantiated treatments no one would have dreamt up by scanning the literature. That provides a new, and truly personal, set of options when conventional measures fail. And if a patient has come to CPCT, a new set of options is what they need most.
Patients flow in and out of the program as their prognoses change. Stephanie Lee, for instance, was presented with new surgical options, and her fly-tested cocktail will now serve as a backup after further validation in a PDX mouse. Cagan and Smibert can’t even say for sure how many patients they’re actively working on, given the fluid state of complex cancer cases; it’s somewhere between four and six. But every patient who ever passes through CPCT leaves a permanent stamp behind.
It’s buzzing in a vial on the 25th floor, brimming with a unique suite of genes that may someday save another patient’s life.
Read Part 1: The Tumor Organoid Biobank
Read Part 3: The Cell Line Critics