Gene Therapy's Next Generation
By Aaron Krol
January 29, 2014 | If you’re in the business of drug discovery, you’ve got to be ready for the long haul. Even small startup companies trying to deliver new treatments will have to pour tens of millions of dollars into research and development over the course of years, only to run the risk of failing in clinical trials and being left with nothing to show for their efforts. One recent study published in Nature Biotechnology suggested that only around 10% of drug indications that enter clinical trials ever reach FDA clearance.
So it takes a special kind of chutzpah to start a company around a type of treatment that has never met with FDA approval. Yet the founders of Editas Medicine, based in Cambridge, MA, are optimistic that now is the time to enter the business of gene therapy – almost a quarter century after the first clinical trials of gene therapy in the U.S. began, without producing a single commercial product to this day. Editas is betting that recent advances in the technology of genetic engineering have leapfrogged previous generations of therapies, opening a huge market niche for a company at the cutting edge of gene editing if it’s game to try its hand at treating genetic diseases.
“That’s what Editas allows us to do,” says Feng Zhang, one of the company’s academic founders. “We can go into the native genome, the natural DNA in the cell, and then make a modification in the genome to correct deleterious mutations.” And they can do it with technologies that didn’t exist when their competitors entered the gene therapy arena.
The classic paradigm of gene editing has been to use an engineered virus to deliver a healthy human gene to a patient with a deleterious mutation. The virus copies its own DNA – stripped of disease-causing material, and including the healthy gene – somewhere into the patient’s cellular DNA, allowing the patient’s cells to produce normal proteins. More recently, zinc finger nucleases (ZFNs) have been used to edit the genome with much greater precision. This technology incorporates Cys2His2 zinc fingers, a class of protein structures that bind to a specific short sequence of DNA. Combining pairs of these zinc fingers with an enzyme that cuts DNA allows researchers to choose a specific stretch of DNA to remove from the genome, and even add a second sequence to replace it. Zinc finger nucleases have the advantages of carving out disease-causing mutations, and being targeted to areas of the genome where inserting new DNA doesn’t run the risk of disrupting genes that are already there; this is the gene editing technology driving companies like Sangamo Biosciences, which currently has two gene therapies in phase 2 clinical trials.
The Technology
Zhang, whose academic lab is part of the Broad Institute of MIT and Harvard, has years of experience working with both ZFNs and the similar TALEN* system. But in early 2011, he read a paper that turned his attention instead to a quirky feature of certain bacterial genomes called CRISPR.
The paper, published in Nature under lead author Josiane Garneau, demonstrated how CRISPR functions as a defense mechanism against bacteriophages – the viruses that attack bacteria. CRISPR stands for “clustered, regularly interspersed short palindromic repeats,” and it was first noticed as a peculiar pattern in bacterial DNA in the 1980s. A CRISPR sequence consists of a stretch of 20 to 50 non-coding base pairs that are nearly palindromic – reading the same forward and backward – followed by a “spacer” sequence of around 30 base pairs, followed by the same non-coding palindrome again, followed by a different spacer, and so on many times over.
Researchers in the field of bacterial immunology realized that the spacers were in fact short sequences taken from the DNA of bacteriophages, and that bacteria can add new spacers when infected with new viruses, gaining immunity from those viral strains. What Garneau and her colleagues showed was the mechanism that made the system work: the spacers are transcribed into short RNA sequences, which a protein called Cas9 uses to find the same sequences in invading viruses and cut the viral DNA at the targeted site.
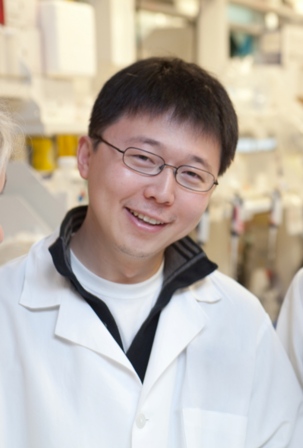
“That was a pretty interesting paper, because it showed Cas9 will cut DNA,” Feng Zhang told Bio-IT World. “And Cas9 uses short RNA sequences to be able to cut the DNA.” Immediately, the system suggested a new method of gene editing: CRISPR-Cas9 complexes could be paired with RNA sequences that target any sites researchers were interested in cutting. The system also had some clear advantages over ZFN or TALEN. Because Cas9 does the cleaving on its own, and carves out an entire stretch of DNA instead of making a single cut, engineers wouldn’t have to combine the system with a cleaving enzyme, or use paired proteins that bind to both ends of the locus they want to modify.
In the fall of 2012, a team including Jennifer Doudna and Emmanuel Charpentier went on to show that CRISPR’s natural guiding system, which features two distinct types of RNA, could be replaced with a single sequence of artificially-produced guide RNA, or gRNA, without compromising its effectiveness. This opened up the possibility of rapid engineering, where only the gRNA sequence would have to be modified to target CRISPR to different areas of the genome. These gRNA fragments would be much easier to engineer than the binding agents of ZFN or TALEN, which use their own elaborate coding schemes to target DNA bases.
Finally, in January 2013, Zhang’s lab published a paper in Science that hit the major benchmark for gene editing: they successfully used a CRISPR-Cas9 system to modify DNA in mammalian cells, both mouse and human. As a flourish, the group encoded multiple gRNA sequences into the same CRISPR array, and showed that Cas9 cleaved out all the relevant sites of the genome. “One advantage of CRISPR [is] you can use it to target multiple genes at the same time,” says Zhang. “And that is actually something that really sets it apart from TALENs or zinc finger nuclease.”
The Company
CRISPR was ready for use creating new strains of model organisms, or in crop engineering, or for any of a hundred important research applications. But from the beginning, the pioneers of CRISPR-Cas9 editing had higher ambitions for the system.
“I thought that maybe we could start a company to use CRISPR to treat genetic diseases,” Zhang says. “And so I went to my boss here at the Broad Institute, Eric Lander, and I asked him if he would be interested in working on starting a company using the CRISPR technology.” Lander recommended a few venture capital firms that specialized in the life sciences and might be willing to take a risk on a game-changing technology in gene therapy – a field that was inherently risky, haunted by past failures, and certainly years away from promising any return on investment.
Three firms – Polaris Partners, Flagship Ventures, and Third Rock Ventures – stepped to the plate, providing $43 million in startup capital in November of 2013 for a company the founding team decided to name Editas. “The type of investing that we do, and the type of company we’re attracted to, is really big idea science and platform technology,” says Kevin Bitterman, a principal at Polaris Partners who is now serving as the Interim President of Editas. “What we really get excited about are the Editas types of technologies: really transformational, broad platforms that can lead to a number of different exciting products.”
Bitterman was part of the early discussions about who belonged in the company and what kinds of disease applications it could tackle. Through Zhang and colleagues at the Broad Institute, a lot of the biggest players in the early development of CRISPR – as well as TALEN, which will also play a role in the company’s research and development – were approached to gauge their interest in tackling gene therapy with these new technologies. “We all decided pretty quickly that it made more sense to band together,” Bitterman told Bio-IT World, “not to form a company in this space, but to form the company in this space.”
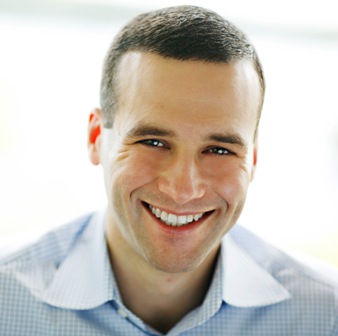
The Editas team of academic founders includes both Zhang and Jennifer Doudna, as well as Keith Joung of Massachusetts General Hospital, and George Church and David Liu out of Harvard. “They’re really the heart and soul of this company,” says Bitterman. “We’re building this company around their science, so they’re much more than advisors… [They’re engaged] in helping think about the indications, in helping us recruit the appropriate people to the company.”
“Many of the scientists that we have hired to get Editas off the ground are coming out of the founders’ labs,” he adds. “They’re the folks that have gotten their hands dirty already with the technology.” Editas is investing heavily in updating its lab space in Kendall Square, and bringing a strong in-house scientific team on board. The founders’ academic labs will continue doing foundational work in making CRISPR-Cas9 ready for therapy, but all the drug development will be Editas’ responsibility.
The company plans to hire 25 to 30 scientific staff in 2014. Their first hire, Morgan Maeder, came from founder Keith Joung’s lab, where she worked with both ZFNs and TALENs, and was first author on a study using CRISPR to activate gene expression in human cells. “She’s worked on all three platforms, and has been working in genome editing for many years as well,” Joung told Bio-IT World. “So when the opportunity came up to join Editas, I think it was a good fit.”
The Challenge
If there’s one thing everyone wants to know about a new gene therapy company, it’s which diseases they think they can cure. There are hundreds if not thousands of genetic diseases that might someday be amenable to gene therapy, most of them vanishingly rare. They affect different tissues, demanding different vectors for drug delivery. They can be caused by a single well-defined mutation, or by many barely-understood ones. For some conditions, the mutated genes will just have to be carved out, while for others they will have to be replaced.
Editas is still considering where its technology can do the most good. “The first thing that we look at is the unmet need,” says Bitterman. “This is potentially such a transformational technology, it’s less exciting for us to go after an area where there’s already therapy and we can potentially make it slightly better… We want to go after areas where there’s truly no other option.”
But practical considerations can make or break any company whose ultimate goal is to get treatments through clinical trials. “We have to be able to deliver Cas9 into the disease-causing tissue,” Zhang points out, “so we have to think about what kinds of delivery systems are available – whether it’s viral, or whether it’s chemical… The second criterion is, what kind of modifications can we achieve efficiently? The most efficient process using CRISPR right now is to knock out a gene. And that means for genetic mutations that have a dominant negative effect, we can use CRISPR to remove that dominant negative gene.” That implies simple Mendelian diseases might be promising first targets for Editas, while polygenic conditions might be further down the road.
Bitterman says that Editas has a prioritized list of possible disease indications, but unsurprisingly, the company isn’t yet ready to name any. There’s a lot of groundwork to be laid before any specific therapeutics go into development. While Editas works to set up a private lab that can tackle therapies, many of the founders are working furiously in their academic labs to make CRISPR-Cas9 specific enough for safe use in humans.
Specificity is a huge concern in gene therapy. A clinical trial in 2003 became a frightening setback for the industry when four infants being treated for severe combined immunodeficiency (SCID) developed leukemia. The virus carrying the corrective gene had inserted its genetic payload into a known proto-oncogene, causing cancerous mutations. Since then, anyone working in gene therapy has been extremely cautious about where the cellular genome is being changed.
CRISPR-Cas9 seems like a promising vehicle for very precise changes to the genome. The gRNA sequences have traditionally been 20 bases long – a convention borrowed from the bacteria that use the CRISPR-Cas9 system naturally – which in theory is more than enough for the targeted sequence to only occur once in the human genome. For comparison, zinc fingers need to be combined in groups of three just to get to nine bases of specificity.
But studies have shown that gRNAs can sometimes lead Cas9 to stretches of DNA that differ from their 20-base sequences by as many as five bases. These off-target hits could have dangerous effects on the cellular machinery, and need to be made vanishingly rare, if not eliminated altogether, before any therapy can go ahead.
This is exactly the sort of foundational science that the founders’ labs can contribute to the Editas project, while also advancing the state of the art for CRISPR-Cas9 users around the world. Just this week, founder Keith Joung published a paper in Nature Biotechnology demonstrating that the specificity of CRISPR-Cas9 can be improved by shortening the gRNA sequences.
“It is totally counterintuitive,” says Joung. “I think most people, including us originally, would think you should just make [the gRNA] longer,” so that more mismatches need to occur before the gRNA will bind to the wrong area of the genome. Yet his lab found that truncated gRNA sequences of 17 or 18 bases, or tru-gRNA, were actually far better at reducing the number of off-target hits, without compromising the ability to hit the real target.
“We could see, in some cases, as much as five thousand-fold improvements or more in the specificity of a truncated guide RNA versus the full-length guide RNA,” says Joung. His working hypothesis is that full-length gRNA has too much binding energy, and that force is enough to overcome a few mismatches. By reducing the number of matches, “we’re reducing the binding energy in the system to a point where it’s just sufficient now for activity… [making] it much more sensitive to any kind of mismatch at the guide RNA-DNA interface.”
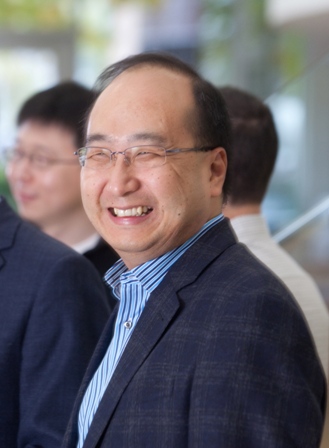
Discoveries like this move the CRISPR-Cas9 system forward, but they also underscore just how young the technology is. Other pushes to improve specificity include using paired nickases: modified Cas9 proteins that only cut one strand of DNA, so that in theory two nickases need to bind to opposite strands before the DNA will be cleaved. But as Joung points out, even measuring specificity is a process full of uncertainty. Current methods, like T7 endonuclease I assays, can only look at one region of the genome at a time, so researchers have to guess where off-target cuts are likely to take place; any unexpected off-target effects will pass undetected.
“What we need next is something that is lacking for all of the gene editing platforms,” says Joung, “which is a method that is unbiased, genome-wide or global in cells, that says where [these off-targets are] occurring.” In the meantime, even by the current, less sensitive measurements, neither tru-gRNA nor paired nickases are enough on their own to bring off-target effects down to zero.
Nevertheless, by gene editing standards CRISPR is moving forward at lightning speed. Less than a year after proving that CRISPR-Cas9 works in human cells, Feng Zhang’s lab was able to produce a library of almost 65,000 gRNA sequences targeting over 18,000 human genes, for in vitro studies of human cells. These resources also fuel future research into CRISPR, as many of the founders take an open science approach to the system’s development. Zhang’s library of CRISPR plasmids is freely available on Addgene, while Joung has been contributing for years to the online resource ZiFiT, a program that helps researchers identify target sites for gene editing with ZFNs, TALENs, or CRISPR; he has already incorporated tru-gRNAs into the ZiFiT software.
“These are broadly applicable technologies,” says Joung, “and I think it’s important to get these tools into the hands of academic researchers who want to use them.” Zhang agrees. “Something like CRISPR is a foundation tool,” he says. “I think making sure these foundation tools are open is important… What my lab has done is try to make the information, the technical know-how, as well as the physical reagents, as accessible to everybody as possible.”
This generous philosophy may seem at odds with building a competitive company, but everyone at Editas seems to agree that their edge has to rest on talent, not on crowding out the field. “Our sense is that this is an important and broad area,” says Bitterman, “and there already are multiple companies working in this area. And we think that’s a good thing. There’s way too many diseases that this can tackle for one company to go after.”
It’s certainly true that there’s more than enough unmet need to go around – if any company is ever able to break through the frozen regulatory environment for gene therapy. There have been some modest signs of a coming thaw, and the Editas team is hopeful that they’ve come along with the best new technology at just the right time for the markets to open up.
“Gene therapy is already used in the clinic,” points out Zhang – just not in the U.S. “In Europe there’s a drug called Glybera, which is a gene therapy product… Especially today, we’re really benefiting from a lot of the hard work that people have done in the past decade.”
“It’s still a very, very new field,” he adds, “so there’s a lot of learning that everybody has to do, and I think a lot of thoughtful discussions need to happen to figure out what is the best way forward. But I think it will definitely change.”
For now, that change is still being outpaced by the transformation in gene editing technologies in the lab. But with each new addition to the arsenal of genetic engineering, a new crop of companies rises up to see whether the right tool is finally available for the job. Given the promise of gene therapy to not just treat some of the world’s most debilitating and intractable diseases, but potentially cure them, we can be grateful that there are still companies like Editas willing to bet that the finish line is finally in sight.
*TALEN stands for transcription activator-like effector nuclease. Like ZFN, TALEN combines an enzyme that cuts DNA with a protein that binds to specific DNA sequences. The same cleavage domains can be used in both systems, but TALEN’s binding domain is a TAL effector, a protein that bacterial species in the Xanthomonas genus use to modify the genomes of the plants they parasitize.